TPJ Editor choice: Getting to the roots of metabolism: the role of fermentation in aerobic conditions in maize root tissues
Highlighted publication: A role for fermentation in aerobic conditions as revealed by computational analysis of maize root metabolism during growth by cell elongation. https://doi.org/10.1111/tpj.16478
Getting to the roots of metabolism: the role of fermentation in aerobic conditions in maize root tissues
Although primary metabolism is highly conserved between plant species, the cells in every tissue have distinct patterns of metabolite fluxes to facilitate different functions. While metabolism is well understood at the cellular level in some leaf tissues, it has not yet been analysed in root tissues. Roots consist of specialized cell types both in the longitudinal direction and across the radial axis. Their primary metabolism provides energy for the uptake of soil resources, functions to biosynthesize macromolecules for growth and to maintain the molecular components of the cells. These functions are not evenly allocated across different cell types, and measuring metabolic flux on a cellular level remains challenging.
Hunt et al. combined a tissue-level cell anatomy model and a cell-level metabolic model of the maize lateral root to predict metabolic fluxes at a tissue scale. First, they used laser ablation tomography to obtain a cellular view of the cross section of a maize lateral root in the elongation zone (Fig.1 A). This was used as basis for RootSlice, a functional-structural model that simulates root anatomy on subcellular to organ scales. They calculated the average change in total volumes of cell components of each torus of cells in the maize root under a specific root elongation rate, and from that the molar rates per hour for total cellulose, phospholipid, and protein biosynthesis. Based on these calculations, they built a flux-balance analysis (FBA) model with cell-torus-specific constraints to reconstruct the potential primary metabolism of each cell torus in the root (Fig. 1B). The model predicted symplastic fluxes of ions and metabolites between cell types, such as of nitrate and sucrose.
Interestingly, the model predicted that substantial amounts of lactate and ethanol, fermentation end products, would be transported throughout the whole root to the epidermis, even though the model was not constrained for oxygen supply. Because fermentation fluxes are related to energy metabolism, the authors compared NAD(P)H /ATP-producing and -consuming reactions. The NAD(P)H/ATP demand was highest in the epidermis, due to the energy cost for proton-coupled uptake of minerals (nitrate and phosphate) from the soil. While the activity of mitochondrial ATP synthase could account for ATP production in the epidermis, it did not account for the ATP produced in the cortex, endodermis, and pericycle. Instead, ATP in these tissues was predicted to be generated mainly by substrate-level phosphorylation in glycolysis. In the absence of oxidative phosphorylation, NADH produced in glycolysis is recycled to NAD+ in a fermentation reaction by lactate dehydrogenases and alcohol dehydrogenase, which explains the accumulation of fermentation end products predicted by the FBA model (Fig. 1C). If fermentation was included in the model, 4 % less enzyme content was required to generate carbon skeletons and energy for cell elongation, suggesting that fermentation also represents an evolutionary advantage under aerobic conditions.
The authors tested this hypothesis by growing maize plants in a continuously aerated nutrient solution, then sampled the elongation zone of roots and analysed the metabolome. They detected substantial quantities of lactate comparable to the levels of other major root metabolites such as hexoses, malate and amino acids, but only a low amount of ethanol. However, ethanol is volatile, and the majority would have been lost to evaporation with the extraction method used. The model predicted that fermentation did not occur in the epidermis, likely because the epidermis had a greater energy demand and a slightly higher demand for the biosynthesis of cell components than the other cell rings. Increasing the energy demand in silico in the non-epidermal cell types or alternating the balance of macromolecule biosynthesis was sufficient to reduce the amount of fermentation predicted. This suggests that the use of fermentation is most efficient in scenarios of low energy use but high requirement for glycolysis-derived carbon skeletons for macromolecule biosynthesis.
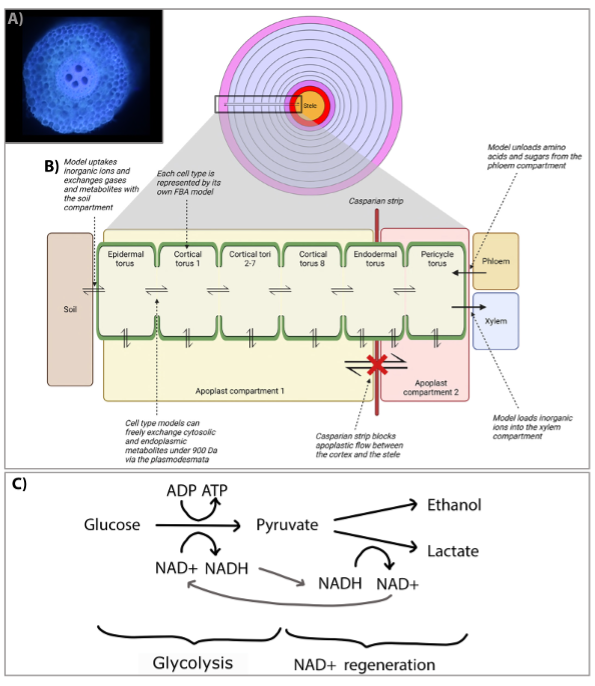
Figure 1: Metabolic fluxes of the maize lateral root analysed by combining a tissue-level cell anatomy model and a cell-level metabolic model.
A) Cross section of the elongation zone of a maize lateral root obtained by laser ablation tomography.
B) Overview of the lateral root metabolic model structure with 11 concentrical cell tori spanning from the epidermis at the soil contact side to the pericycle adjacent to the vasculature.
C) Fermentation recycles NADH for use in glycolysis. A, B): modified from (Hunt et al., 2023).